Prelude
When art meets science, revelations unfurl in the most unexpected ways. In 2006, physicist José Luis Aragon and his collaborator Manuel Torres visited the Thyssen-Bornemisza National Museum in Madrid, where Vincent Van Gogh’s swirling Starry Night sparked a question: could the turbulence in the sky be expressed mathematically? This casual musing at a rooftop café led to groundbreaking research, linking Van Gogh’s brushstrokes to Russian mathematician Andrey Kolmogorov’s laws of turbulence. Turbulence, a chaotic phenomenon governing fluid dynamics, remains one of science’s greatest mysteries. Yet, through his masterful manipulation of brightness and colour, Van Gogh unwittingly captured its essence. By statistically analysing the luminance in his work, researchers discovered patterns akin to energy cascades in natural vortices. Starry Night became more than art — it embodied a scientific truth.
This story weaves through centuries of inquiry, from Japanese artist Katsushika Hokusai’s fractal waves to the energy eddies studied by modern physicists, demonstrating how artists and scientists, through different lenses, unravel the profound mysteries of nature.
Every April, José Luis Aragon would spend time with his friend Manuel Torres in Madrid, Spain. Aragon, the director of the Centre for Applied Physics and Advanced Technology in Mexico, and Torres, a scientist at the Spanish National Research Council, were not only friends but also research collaborators.
During this time of year, a lingering winter, like a carelessly discarded blanket, clings to the city. The entire city bursts into colour, adorned with roses, tulips, daffodils, cherry blossoms and wisteria. The leaves of the trees, brimming with vibrant green, seem as if they were meticulously painted by an artist who had toiled through the night. The city comes alive with fairs and exhibitions, pulsating with a festive spirit. Madrid’s rooftop cafes, restaurants and bars overflow. The clamour of the city blends with the clinking of spoons in coffee cups and the lively rhythms of jazz, pop and guitar. The air is filled with the fragrance of flowers, fresh spring leaves, freshly brewed coffee and the tang of beer from the local pubs, creating a unique olfactory experience on the streets of Madrid.
The two friends had co-authored numerous research papers. Thus, their time together was filled with both leisure and scholarly discussions. Torres was passionate about art, particularly painting and plastic arts. On the other hand, José loved music. However, both shared an immense love for literature. And both enjoyed visiting museums to admire art.
On one such April evening in 2006, the two friends visited the Thyssen-Bornemisza National Museum, which housed several works by the Dutch artist Vincent Van Gogh. While admiring Van Gogh’s 1890 painting, Les Vessenotsen Auvers or Landscape of Auvers, Torres wondered if it were possible to express in mathematical terms the kind of turbulence or wave-like patterns that Van Gogh created with his brushstrokes.
Aragon initially doubted the feasibility. On their way back from the museum, while sipping drinks at a rooftop cafe, they resumed their discussion on the topic. It was at that cafe that they decided to research Van Gogh’s most famous painting Starry Night.

It was on the 18th or 19th of June, 1889, that Van Gogh gave the final touches to Starry Night. He informed his brother Theo of this the following day. At the time, the painting was not particularly appreciated but today it is priceless; its popularity is unmatched. Nearly three million people visit the Museum of Modern Art in New York, US,to see the painting each year. If there were a single word to perfectly describe it, it would be “iconic”. Starry Night has reached every aspect of life: from living rooms to coffee mugs, from t-shirts to wall graffiti, from diary covers to necklaces and earrings. Beyond this all, its popularity extends even to the world of modern science. Not only artists and art lovers but also many scientists are fans of this work of art.
While most viewers are drawn to the swirling sky with its eleven stars and turbulent clouds in the background, the foreground of the painting often goes unnoticed. The waves of white clouds crash across the canvas from one end to the other. In the upper right corner, a crescent moon, the colour of a lemon peel — a blend of zinc yellow and Indian yellow — is surrounded by a radiant halo. The star near the horizon is unusually large, just as Van Gogh saw it in his dreams. (I have a problem in understanding this bit. It would seem both the background and foreground are the clouds. The foreground of the painting is the cypress tree; this has to be clarified. And why it goes unnoticed.)
Through the vibrant use of colours and brushstrokes, all the elements of the painting – the stars, the clouds, the waning moon – seem to be in motion, almost alive. They pulsate with a vital energy that anyone who sees the painting can feel. This is what makes Van Gogh unique. Unconsciously, Van Gogh seems to have created variations in colour, bringing the entire canvas to life and making it dynamic. Researchers have converted a digital version of the
painting into black and white. They found that Van Gogh created turbulence by varying the luminance or intensity of light emitted per unit area.
Finding Pattern
Once they began their research, Torres and Aragon found themselves in uncharted waters. They were unsure of how to proceed. Then, Aragon came across a book by neurobiologist Margaret Livingstone titled Vision and Art: The Biology of Seeing. It was from this here that Aragon learned about how the human brain processes the colour and brightness of an object in different areas. Information about the colour and shape of an object is stored in the ventral pathway of the brain, while information about the object’s movement and changes in motion are processed in the dorsal pathway. Livingstone writes in her book that this technique has been used in painting for a long time.
According to this technique — called equiluminance — the brightness of light is maintained as almost uniform throughout the canvas, while the colours are in contrast. This creates an optical illusion, making the brain perceive different areas even though the brightness is the same. As a result, these areas may appear blurry or flickering.
This suggested that there was a connection between the variations in the light emitted from an object and its perceived movement. Whenever there is a change in the brightness or colour of light, our brain assumes that the object is in motion.
Torres and Aragon then decided to conduct a statistical analysis of the brightness of colours in some of Van Gogh’s paintings to identify the characteristics of the swirling patterns.
Turbulence is a ubiquitous phenomenon in nature, whether it’s in water or air. The tiny particles in air, water, smoke or any other fluid are constantly moving in different directions. This random and chaotic motion suddenly begins to swirl in a curved fashion, creating a new pattern: a vortex.
We can see vortices in the water swirling around a boulder in a river, in the smoke from a cigarette, in the wind flowing over an airplane wing, in milk being churned into coffee, in the wind blowing through the branches of a tree, in ocean currents and even in the blood flowing through our veins. Despite its ubiquity, the cause of turbulence remains one of the most challenging mysteries in science.
Nobel laureate physicist Werner Heisenberg once remarked, “When I meet God, I am going to ask him two questions. Why relativity? And why turbulence? I really believe he will have an answer for the first.”
In 1923, Werner Heisenberg’s PhD thesis explored the complex transition from smooth (laminar) to chaotic (turbulent) fluid flow. He aimed to pinpoint the moment this shift occurs, a notoriously difficult problem in fluid dynamics. Heisenberg tried to understand the conditions under which a smoothly flowing fluid would lose its stability and transition into a turbulent state. He developed a mathematical model to describe the behaviour of small disturbances within a flowing fluid. Due to the immense complexity of the problem, Heisenberg provided an approximate solution. His model offered insights into the factors that influence the onset of turbulence, such as fluid velocity and viscosity.
Although the origin of turbulence remains a mystery, Russian mathematician Andrey Kolmogorov provided a framework for understanding its behaviour. Kolmogorov’s theory explains how energy flows from larger eddies to smaller ones, creating a continuous cascade of energy dissipation. This entire process, as demonstrated by Kolmogorov, adheres to the law of conservation of energy. As a fluid transitions from larger to smaller eddies, its motion changes. Smaller eddies rotate faster. The total energy remains the same in each eddy. The process is called energy cascading. Despite the complexity of these turbulence patterns, Kolmogorov discovered remarkable statistical similarities, simplifying our understanding of turbulence to some extent.
This brings to mind British mathematician Lewis Fry Richardson’s poetic description of the nature of turbulence:
Big whorls have little whorls
Which feed on their velocity,
And little whorls have lesser whorls and so on to viscosity.
Aragon and Torres analysed the digital copy of Starry Night and discovered that the brightness of light in the painting corresponded to the energy cascade described by Kolmogorov. In other words, they found the same pattern in the flow of light energy. They observed that the swirling patterns in the sky of Van Gogh’s masterpiece followed the same rules as those in real fluids.
Aragon pointed out that the French painter Claude Mone had pioneered this technique in his 1872 masterpiece, Impression, Sunrise. Monet, a key figure in the Impressionist movement, sought to capture the fleeting effects of light and atmosphere. He achieved this by applying small dabs of pure colour directly to the canvas, thus creating a shimmering, almost vibrating effect. This technique, where the viewer’s eye blends the colours to create an overall impression, is a hallmark of Impressionism. Aragon observed that Monet, by manipulating the interplay of light and colour, created a sense of movement and dynamism in his paintings, much like the turbulent flow of a fluid. This technique provided a crucial link between art and the scientific understanding of motion and perception.
Van Gogh’s sky was turbulant
Science, however, does not stand still. This controversy (proposition would be more suitable; there isn’t anything here yet to say the matter was contested) caught the attention of François G. Schmitt, a physicist at the French National Centre for Scientific Research (CNRS), Grenoble, and a co-author of the research paper (Please mention the title). In April 2024, Schmitt met with his former student Yongxiang Huang of Xiamen University in China. Together, they decided to revisit the mathematical validity of the turbulence in clouds in Van Gogh’s famous painting.”*
The researchers obtained a digital copy of Starry Night and converted it to grayscale. They then isolated the swirling cloud formations, removing all other elements unrelated to the flow.
Upon analysis, they discovered that the luminance of the swirling clouds followed a pattern similar to that of energy flow. Just as the speed of particles changes as a fluid transitions from larger to smaller eddies, the energy emitted from the colours in the painting’s turbulent clouds also changed in a swirling pattern. The size, flow and intensity of the swirls in Starry Night adhere to the same physical laws that govern turbulence in flow.
In essence, this new study further solidified the research conducted by Aragon and Torres in 2008. This mathematically enriched study provided compelling evidence that Van Gogh had indeed captured a natural phenomenon with his fine strokes. Starry Night follows the same physical laws that govern turbulence in flows. The paper (name, please) suggests that Van Gogh may have closely observed real-life fluid movements. Schmitt and his team did not limit their analysis to just Starry Night. The researchers also analysed Chain Pier, Brighton, a landscape painting by British artist John Constable, and the image of Jupiter’s Great Red Spot captured by Voyager 1 (1979). In both of these, they found turbulence-like patterns that support Kolmogorov’s theory.
However, a recent study by Mohamed Gad-el-Hak and James J. Riley, argued that that the earlier paper’s findings are fundamentally flawed. They point out that the Kolmogorov theory—originally developed for fluid velocity fields and later extended by Obukhov and Corrsin to scalar fields like temperature or pressure—was wrongly applied in the earlier Physics of Fluids study.
The study says there’s no measurable scalar property in the painting to justify using that theory, and the assumed atmospheric flow doesn’t meet the theory’s basic requirements either.
And so, the debate goes on. But what’s truly remarkable is how a single painting has inspired scientists for decades to explore the very nature of turbulence. You need an Artist’s eye
Nature isn’t easily understood. It reveals itself to those who observe it deeply and seek to comprehend it with passion. Often, scientists overlook details that artists readily perceive. The swirling patterns of fluids and gases are one such phenomenon that captivates the creative mind. While mathematics, particularly the complex Navier-Stokes equations, can provide some understanding of these vortices, their transformative nature extends beyond the realms of physics and mathematics, touching upon philosophy, psychology and performance art. (Would help to put in a bit about the equations; as a reader I feel there’s a gap now that they have been mentioned)
The story of these equations goes back a long way. Mathematicians and physicists like Leonhard Euler and Claude-Louis Navier started working on them in the 1700s and 1800s. Then in the mid-1800s, Irish mathematician and physicist George Gabriel Stokes made some important improvements, giving us the equations we use today.
Even though they’re crucial, the Navier-Stokes equations are still a big mystery for mathematicians. They’re one of the seven Millennium Prize Problems, which means solving them would be a huge breakthrough in mathematics.
So, while they might sound complicated, these equations are essential for understanding the world around us. They help us predict how fluids behave, which is crucial for many aspects of
our lives.– from how weather patterns change to how blood flows through our bodies. Engineers use them to design airplanes, ships and even pipelines. They’re also crucial for weather forecasting models.
At the University of Melbourne, Australia, scientist Andrew Melatos, artist Briony Barr and a group of students embarked on a novel approach to visualise turbulence. They created a large glass chamber containing two large fans and several Styrofoam balls. When the fans were turned on, the balls began to swirl within the airflow, creating intricate patterns. They named this exhibit “A Hierarchy of Eddies”. Initially, only one fan was activated, causing the balls to form gradual swirling patterns. Upon activating the second fan, these patterns became incredibly chaotic and dynamic, creating a constantly evolving visual spectacle for the audience. This exhibit was part of the long-running ‘Experimenta Make Sense: International Triennial of Media Art” exhibition. In this event, visitors become active participants. Each viewer discovers unique patterns, resulting in personalised experiences that blur the lines between art and science. Everyone becomes both an artist and a scientist, engaged in the process of exploration.
The same pattern of turbulence that creates tornadoes and typhoons is also involved in the formation of raindrops, the control of drag in cars, planes and submarines, and even the swirling air caused by a hummingbird’s wings. On a cosmic scale, similar vortices are created when galaxies collide. However, the chaotic nature of turbulence makes it extremely difficult to accurately predict. No two turbulent flows are identical. Their patterns never repeat. When weather forecasts are inaccurate, we often blame meteorologists but we fail to realise the immense complexity of the problem.
Thousands of research papers have been published on turbulence but most are observational. This indicates that significant progress in solving this problem has yet to be made. Other scientific fields have advanced so rapidly that a scientist returning to a field after twenty years might find it unrecognisable. However, a fluid dynamics researcher would have no trouble understanding the subject. This stagnation suggests that we need to broaden our perspective.
Artists who have been observing turbulence through different lenses for centuries may contribute to this understanding. They are drawn to turbulence not because of its practical implications or complex challenges but because of the beauty and drama it brings to natural phenomena like clouds, waterfalls and stormy seas. From this unique perspective, artists offer a complementary viewpoint to the world of physics.
The wave
Some historical paintings and illustrations have vividly depicted the structure of turbulence, a fact that scientists have recently acknowledged. A striking example is the famous woodblock print The Great Wave off Kanagawa by the Japanese artist Katsushika Hokusai, which beautifully showcases the fractal nature of turbulence. It is known that Van Gogh was greatly influenced by Hokusai’s art. Art historians believe that Van Gogh was an avid collector of Japanese prints and was particularly fond of Hokusai’s woodblock print, which is now a widely recognised piece of art. In a letter to his brother Theo, Van Gogh wrote, “These waves are claws, the boat is caught in them, you can feel it.”
In a thought-provoking paper, physicist Julyan Cartwright from the University of Granada, Spain, and Hisami Nakamura from Chuo University, Tokyo, discuss Hokusai’s artistic approach: [Hokusai] focuses on a single aspect of what he observes, deliberately omitting extraneous details. While a photograph can be stunning, realistic, and richly detailed, it merely reproduces nature without offering deeper insights into the captured scene. Hokusai, however, adopts a different perspective in his art. By removing superfluous elements that might distract
the viewer, he highlights the profound and essential features of a scene, inviting us to see and appreciate it in a new and transformative way.
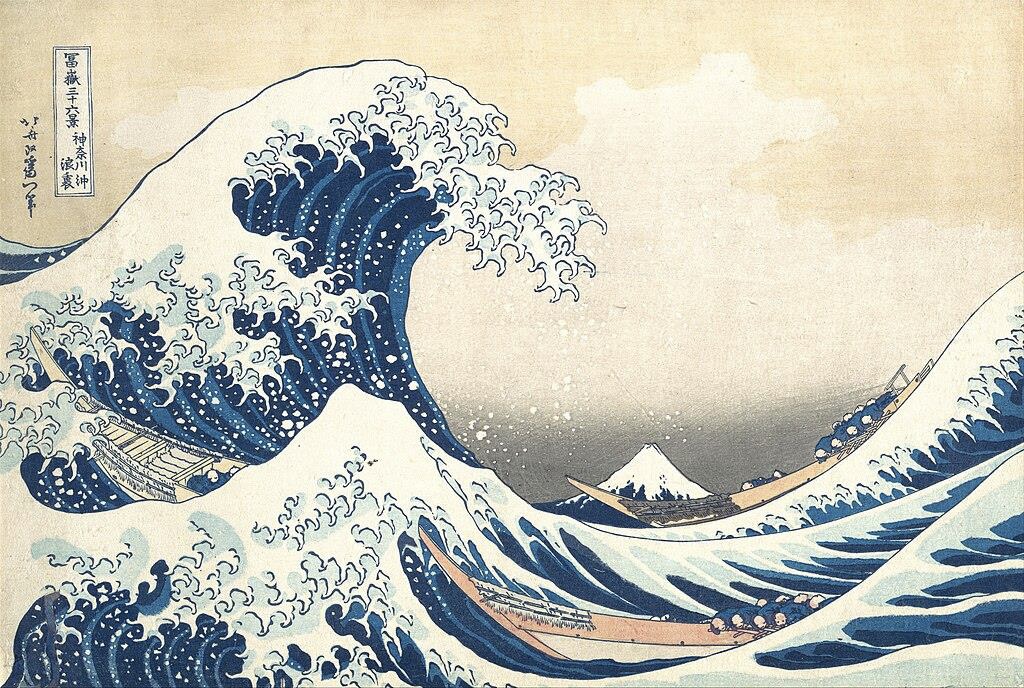
Flow visualisations have both advanced and complicated the study of turbulence. Photographic images often contain an overwhelming amount of information. Data on velocity fluctuations, gathered using a hot-wire anemometer, offer a clearer picture of turbulence (left). Analysing this data with a correlation function reveals how eddies of varying sizes decay over time (right). The resulting curve helps scientists interpret the distribution of scales and their significance in turbulence in flow.
Zellman Warhaft, a former professor at Cornell University, US, dedicated forty years of his life to studying how turbulence arises from the flow of air within tunnels. In a November 2022 article for American Scientist, he stated, “An artist sees with an insight that a scientist often misses. By combining the perspectives of both, we can make significant strides towards a deeper understanding of turbulence.” In his article, Warhaft mentions that Painter David Hockney once remarked, “Van Gogh saw the landscape very clearly. Most people scan the ground to see where to walk, but they don’t really look. Van Gogh looked.” Great artists observe the world in a unique way, reshaping how we perceive it. After seeing Van Gogh’s irises, we do not view the flowers in the same way again — he changes the way we see.
Fluid mechanicians share this distinct way of seeing. Like artists, they emphasise certain features while downplaying others to better understand their subject. While their methods differ, scientists can draw inspiration from artists. Historically, painters and illustrators have often outpaced researchers in capturing the nuances of fluid flow. Contemporary artists like Gerhard Richter and Vija Celmins create hyper-realistic depictions of the sky and water that transcend literal representation, offering fresh insights into nature. Perhaps blending artistic vision with modern flow visualisations and simulations can deepen our understanding of turbulence.
After his retirement, Warhaft took up painting, focusing on water waves and vortices. This was, in a sense, a continuation of his research, as he sought to capture the essence of turbulence that had eluded him for decades.
From Leonardo da Vinci to contemporary artists like Gerhard Richter, Sigmar Polke and Ned Kahn, many have attempted to visualise turbulence. However, Van Gogh’s case was unique. He never actually witnessed such celestial turbulence in the night sky. Psychologists suggest that Van Gogh’s psychological turmoil may have influenced his perception of nature, leading him to paint these turbulent scenes.
But let’s leave that aside for now. Instead, let’s turn to the words of musician Don McLean in his song Vincent:
Starry, starry night
Flaming flowers that brightly blaze
Swirling clouds in violet haze
Reflect in Vincent’s eyes of China blue
Colours changing hue
Perhaps it would make sense to listen to the entire song.