Marc lounged comfortably in his favorite armchair, savoring the rich bitterness of his afternoon espresso. The sun slanted through the café’s lofty windows, casting warm pools of light across the polished wood floor. Just then, Amanda burst in, her eyes bright with excitement—so bright that she skidded on a stray floor mat and sent Marc’s cup careening. Dark coffee arced through the air and splattered across his crisp white shirt.
A tense beat passed. Steam curled from the ruined espresso, and Marc glanced at the spreading stain. Amanda’s cheeks flushed; she braced for an outburst. But instead of anger, laughter bubbled between them—an unexpected spark that dissolved any hint of blame.
“What do you say,” Marc grinned, “we follow this stain down the rabbit hole?”
Stories of Stains and Patterns Around Us
And so began their curious odyssey through the hidden beauty of blemishes. They left behind the predictable world of spotless perfection and stepped into a realm where spills, smudges, and smears revealed secret patterns and surprising elegance.
Their first discovery came on Marc’s shirt: the stain’s edges unfurled like delicate lace, each droplet capturing a microscopic landscape of rippling contours. Under a café light, they examined how the coffee’s tannins spread, subtly shifting from deep espresso brown at the center to pale amber at its fringes. What at first seemed a random blot now glimmered with fractal complexity.
Outside, on the cobblestone street, Amanda pointed to a rusty water stain curling beneath an old drainpipe. It formed concentric rings, as orderly as tree rings in a centuries-old oak. Each ring marked a moment when rainwater pooled and receded, leaving behind a thin mineral outline that turned decay into art.
Further on, in a sunlit park, their journey led them to a discarded polka‑dotted scarf, its fabric mottled with splashes of tea and ink. Amanda ran her fingers along the stains, tracing tiny constellations of color that mapped out the scarf’s hidden adventures—picnics, cafe tables, handwritten letters sipped alongside strong brews.
Every stain told a story, and every smudge held a secret beauty. In peeling paint, spattered mud, and even in the patterns of spilled wine on a dinner table, Marc and Amanda discovered nature’s wild choreography: the dance of fluids and solids, the interplay of gravity and surface tension, the poetry in imperfection.Their adventure hadn’t begun with a plan—it began with a spill. And through that accident, they unearthed a lesson: that even life’s little messes can unveil astonishing beauty if we only pause to look.
“What is life if full of care, if we have no time to stand and stare?” Chimed Marc quoting W. H. Davis! Do you have a moment to stare deeply at the science of stains and patterns? If yes, then let’s arch back to the beginning!
Of Colloids and Coffee Ring Effect
Pouring or spilling a beverage onto your shirt can yield different results depending on the drink’s microscopic makeup. Take green tea: if a few drops land on a white shirt, you’ll usually blot them up and find almost no trace of color once the fabric dries. That’s because green tea is a true solution of small, water-soluble molecules — polyphenols and tannins that remain fully surrounded by water molecules. In solution, these individual molecules are too tiny to scatter light or to hitch a ride on evaporative flows. Hence, there’s no mechanism to concentrate them at the edge of a drying droplet. In other words, with nothing to carry them outward, you won’t see a “coffee‑ring” stain pattern, and any faint coloration washes away easily.
Coffee, on the other hand, is a colloid: microscopic particles of oils, melanoidins, and caramelized sugars suspended in water. When a coffee droplet evaporates, liquid is lost fastest at the perimeter. To replace that evaporating fluid, capillary flows sweep liquid (and its suspended solids) from the center toward the rim. As evaporation continues, those particles accumulate in a dark, ring-shaped deposit — the hallmark coffee‑ring effect. That stubborn brown outline is what clings to fabric fibers when you spill coffee on a shirt, leaving a telltale stain.
Red wine sits somewhere between these two extremes. Although its alcohol content might suggest a simple solution, red wine actually behaves like a colloid as well. Tiny aggregates of anthocyanin pigments, polyphenols, and tannins — sometimes bound to proteins or polysaccharides — float in the liquid. As you sip the last drops, you’ll often notice a faint ring of reddish‑brown residue around the bottom of the glass. This is the same coffee‑ring phenomenon in action: evaporation at the glass wall draws fluid outward, depositing those pigment aggregates in a circular band.
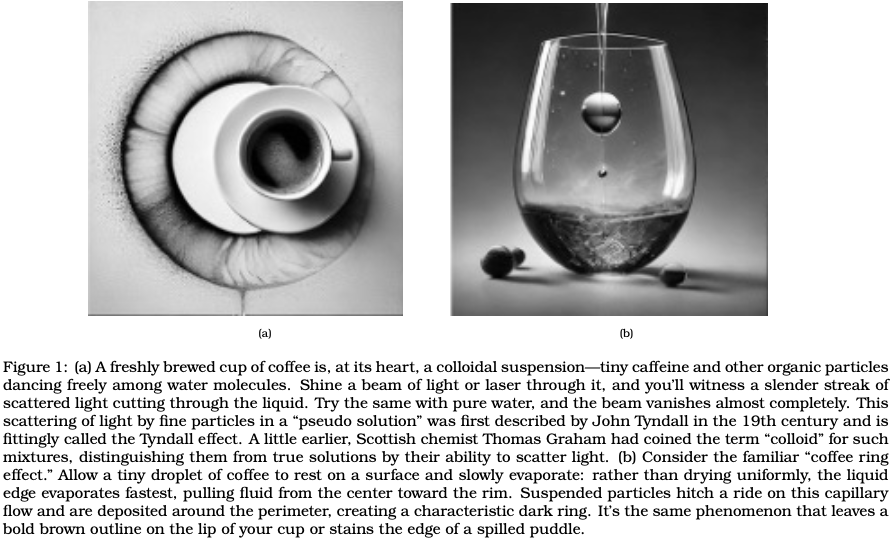
But red wine offers yet another visual surprise: the “tears” or “legs” that climb up the glass walls. Here, alcohol evaporation plays a starring role. Alcohol has a lower surface tension than water; when it evaporates more quickly near the meniscus, it creates a surface‑tension gradient that pulls liquid up the glass in a thin film. Gravity eventually overcomes that pull, and droplets run back down, tracing elegant streams of wine. This Marangoni‑driven convection underscores how subtle differences in composition—water vs. alcohol, dissolved vs. suspended — shape the fluid’s motion and its residues.
So, if you spill red wine on a white shirt, you get a stain more akin to coffee than tea. The suspended pigment aggregates follow capillary flows to the droplet’s edge, embedding in the fibers and leaving a deep‑colored ring or blotch that resists washing. In contrast, green tea’s fully dissolved molecules don’t concentrate during drying, so little to no visible stain remains. In summary, whether a spill vanishes or becomes a permanent mark depends on the drink’s colloidal nature and the interplay of evaporation, capillary flow, and surface‑tension gradients. Green tea — a true solution — leaves virtually no stain. Coffee and red wine—both colloidal suspensions — give rise to characteristic rings as particles migrate outward. And only wine, with its alcohol, creates climbing “tears” before it settles back as another testament to fluid dynamics elegantly written in your glass.
Unleashing the beauty of butterflies and opals from Coffee rings
The delicate brown ring left by a drying drop of coffee is more than a stubborn stain—it’s a miniature laboratory of nature’s self-assembly. As a droplet evaporates, liquid vanishes fastest at its edges. To replenish that loss, capillary flows sweep microscopic coffee particles outward, depositing them in a neat, circular band. Even more subtle forces at work—like tiny gradients in surface tension, known as Marangoni flows — can fine-tune how particles dance toward the rim. The result is a spontaneously emerging pattern that needs no external guidance: a self-assembled structure born from the interplay of evaporation, capillarity, and intermolecular forces.
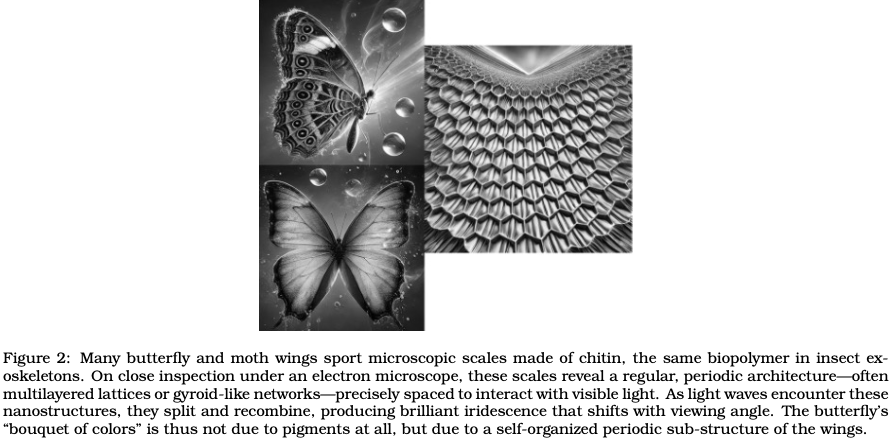
These “coffee rings” exemplify self-organization, where simple local interactions give rise to complex, ordered structures. Each sedimented particle follows basic physical laws — evaporative flux, adhesion to the substrate, Brownian motion—yet collectively generates a striking macroscopic design. In many ways, this is the heart of self-assembly: building blocks (colloidal particles, molecules, or nanoparticles) find their places through mutual forces — electrostatic attractions, van der Waals forces, steric hindrance — without any central coordinator. Such emergent architectures appear across chemistry, biology, and materials science, wherever components can move, interact, and lock into place.
One of the most beautiful realizations of this principle hides within opal gemstones. Natural opals consist of silica nanospheres—tiny, uniform particles just a few hundred nanometers across—that have organized into a three-dimensional close-packed lattice. As water slowly evaporates from the gel of silica and mineral precursors, the spheres settle into highly ordered arrays. Once dried and solidified, this periodic arrangement behaves like a photonic crystal: incoming light waves interfere constructively at specific wavelengths, scattering vibrant flashes of reds, greens, blues, and every hue in between. What you perceive as the opal’s “play‑of‑color” is this structural coloration—the gemstone’s internal lattice diffracting and reflecting light in a symphony of rainbow hues.
Remarkably, the same self-assembled photonic structures appear in the natural world with living materials. Many butterfly and moth wings sport microscopic scales made of chitin, the same biopolymer in insect exoskeletons. On close inspection under an electron microscope, these scales reveal a regular, periodic architecture—often multilayered lattices or gyroid‐like networks—precisely spaced to interact with visible light. As light waves encounter these nanostructures, they split and recombine, producing brilliant iridescence that shifts with viewing angle. The butterfly’s “bouquet of colors” is thus not due to pigments at all, but to the same physical phenomenon that makes opals glow.
From coffee stains to opal gemstones to butterfly wings, the principle is consistent: given the right ingredients and conditions, tiny building blocks can organize themselves into complex, functional architectures. In the coffee droplet, gravity, evaporation, and surface forces choreograph colloidal particles into a ring. In geological formations, silica spheres gradually pack into crystalline arrays. In living organisms, biological molecules assemble with nanometer precision to manipulate light for warning signals, camouflage, or mate attraction. All these examples remind us that order need not be imposed from above—sometimes, the simplest interactions at the smallest scales weave the most exquisite patterns in our world.
Epilogue
As a butterfly lifts itself from a moss‑clad crevice by the roadside, Marc’s coffee stain has settled into russet tones, echoing nature’s own palette. Amanda reaches out to brush its delicate wings, as though yearning to graze the very folds of Zeus’s robe — the divine tapestry of form and pattern. Beyond them, the ocean’s roar sculpts the shoreline into ever‑shifting fractals, each wave drawing new Mandelbrot arcs in the sand. Overhead, drifting gray clouds catch flecks of sunlight that dance in harmony with the butterfly’s iridescence and the lingering aroma of espresso. In that convergence of wing, wave, and stain, Marc and Amanda find themselves drawn toward the horizon — walking side by side into a boundless conversation about structures: built by nature, inspired by science, and woven into every surface they touch.
Further Reading
- Deegan, R. D.; Bakajin, O.; Dupont, T. F.; Huber, G.; Nagel, S. R.; Witten, T. A. Capillary Flow as the Cause of Ring Stains from Dried Liquid Drops. Nature 1997, 389, 827–829.
- Deegan, R. D. Pattern Formation in Drying Drops. Phys. Rev. E 2000, 61 (1), 475–485.
- Hu, H.; Larson, R. G. Marangoni Effect Reverses Coffee Ring Depositions. J. Phys. Chem. B 2006, 110 (14), 7090–7094.
- Yunker, P. J.; Still, T.; Lohr, M. A.; Yodh, A. G. Suppression of the Coffee Ring Effect by Shape Dependent Capillary Interactions. Nature 2011, 476, 308–311.
- Sempels, W.; De Dier, R.; Ryckelynck, M.; De Buysser, K.; Hofkens, J.; Vermant, J.; Ramon, H. Auto Production of Biosurfactants during Evaporation Induced Self Assembly: How Bacteria Make Dried Puddles Dance. Soft Matter 2013, 9, 1712–1717.
- Park, J.; Moon, J.; Shin, S.; Wang, D.; Mariella, R., Jr.; Kim, C.-J Control of Colloidal Particle Deposition in Evaporating Sessile Droplets. Langmuir 2006, 22 (8), 3506–3513.
- Tang, X.; Liu, D.; Sun, C.; Zeng, H. Evaporation Dynamics and Deposit Morphology of Tea Droplets with Surfactants. Colloids Surf., A 2017, 529, 59–67.
- Vukusic, P.; Sambles, J. R.; Lawrence, C. R.; Wootton, R. J. Quantified Interference and Diffraction in Single Morpho Butterfly Scales. Proc. R. Soc. Lond. B 1999, 266, 1403–1411.
- Vukusic, P.; Sambles, J. R. Photonic Structures in Biology. Nature 2003, 424, 852–855.
- Kolle, M.; Lee, S.; Chae, E. J.; Vukusic, P.; Spearing, S. M.; Steiner, U. Neotropical Butterflies Exhibit Highly Effective Photonic Nanostructures. Adv. Mater. 2013, 25, 2231–2236.
- Kinoshita, S.; Yoshioka, S.; Miyazaki, J., Physics of Structural Colors. Rep. Prog. Phys. 2008, 71 (7), 076801.
- Truscott, T. T.; Stevens, G. W.; Smith, M. E. The Coffee Ring Effect: Fundamental Mechanism and Control Strategies, Adv. Colloid Interface Sci. 2020, 274, 102045.
- Parker, A. R.; Townley, H. E. Biomimetics of Photonic Nanostructures, Nat. Nanotechnol. 2007, 2, 347–353.